For stars, there is life after death. In an average star, when most of its hydrogen has fused into helium and helium into other heavier elements, it lazily swells, expelling vast amounts of material back into space. This is how our Sun will end—not with a bang but with a whimper—leaving behind a very small but dense core called a white dwarf.
The mass of a star determines its fate. For very massive objects, above eight times the mass of the Sun, when the fuel in the core is exhausted, the tremendous gravity becomes its atoms’ biggest enemy. The electrons plunge against the nuclei, weakly reacting with the protons producing neutrons.
Neutron stars
If the core has 1.4 times the mass of the Sun (called the Chandrasekhar limit), in one-millionth of a second, it shrinks from the size of Mars into a ball—mostly made out of neutrons—the size of a Manhattan (between ten to twenty kilometers across). Stars with such cores are called neutron stars.
During this process, their outer layers bounce out in a spectacular supernova, ejecting elements created in the star and heavier elements than iron synthesized right at the moment of the explosion. If the core is under three solar masses, it will remain a neutron star. Above three solar masses, it will keep shrinking until it becomes a black hole.
Neutron stars are far from quiet, dark objects. They rotate incredibly fast, launching jets of particles and radiation into space, seen from the Earth as cosmic lighthouses scientists call pulsars.
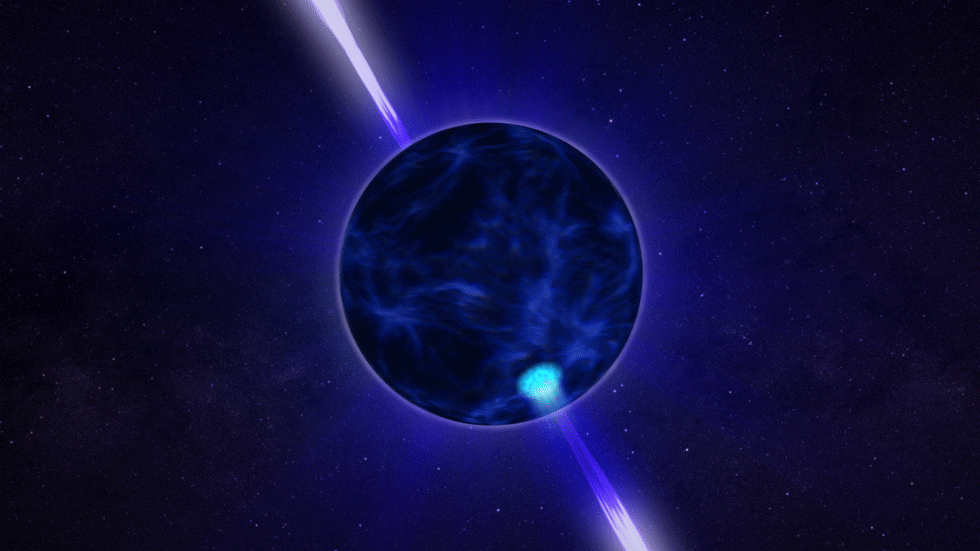
A sphere ma non troppo
Because of their enormous gravitational pull—a billion times stronger than that of the Earth—every feature on a neutron star’s surface should be squashed to minuscule dimensions, which means that a neutron star should be close to a perfect sphere. To grasp how dense these objects are imagine the mass of Mount Everest fitted in a tablespoon.
General relativity predicts that a spinning neutron star emits traveling ripples of space-time, known as gravitational waves, provided they have deformities on their surface.
Now that we have entered the era of gravitational waves astronomy, scientists have the potential to find out whether neutron stars have imperfections or “mountains” on their surface.
Fabian Gittins and Nils Andersson from the University of Southampton in the UK simulated the forces that may cause imperfections in neutron stars. They are trying to find out how these hypothetical mountains would have emerged and how large they would be. The scientists modeled the highest peak a neutron star crust can support, i.e., before the crust fractures. Such knowledge provides limits to the strength of the gravitational waves a neutron star would produce.
Newton: zero, Einstein: one?
Gittins and Anderson introduced Einsteins’ gravity and compared their results to previous calculations where scientists used Newtonian gravity. In this new study, they also introduced surface forces and thermal pressure. According to their results, the largest mountains in a fast-spinning neutron star (about one kilohertz) should be a fraction of a millimeter tall, one hundred times smaller than previous estimates.
“In reality, the force is related to the formation history of the star, which may involve complex mechanisms like quakes and accretion from a companion. Therefore, in order to get a handle on the force, evolutionary calculations that consider the history of neutron stars will be necessary. It should be noted that such calculations would need to be ambitious in order to take into account the physics that may be important in the evolution of the crust, such as cooling, freezing, spin down, magnetic fields, and cracking (to name but a few mechanisms).”
Elusive gravitational waves
Since the Laser Interferometer Gravitational-wave Observatory started to detect gravitational waves in 2015, they have observed the merging of rotating black holes, the collision of two neutron stars, and a black hole swallowing a neutron star. Gravitational waves arising from a single rotating neutron star have not yet been observed.
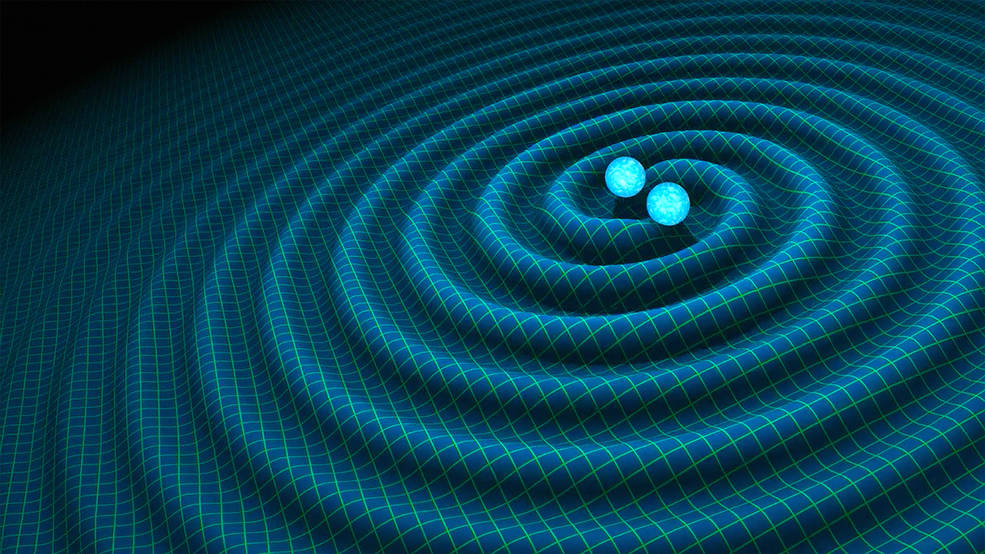
According to Gittins and Anderson, the smaller the mountains on neutron stars are, the smaller are the gravitational waves they produce. That might be the reason for the lack of detection. However, scientists are optimistic:
“There is hope on the horizon that we will soon detect gravitational waves from rotating neutron stars for the very first time,” the authors wrote in their paper accepted for publication in Monthly Notices of the Royal Astronomical Society. Innovations in gravitational waves detection would shed light on the size and existence of mountains on neutron stars.
Investigating supermassive cosmic objects like neutron stars pushes the frontiers of quantum physics and general relativity. Neutron stars are definitely a great “laboratory” to study quantum gravity. But that is a story for another time.
References
F. Gittins and N. Andersson. (2021). Modelling neutron star mountains in relativity. Eprint 2105.06493. Accepted for publication in Monthly Notices of the Royal Astronomical Society.
Morgan Hollis. (2021). A bug’s life: millimetre-tall mountains on neutron stars. Royal Astronomy Society, press news.
F Gittins, N Andersson, D I Jones. (2021). Modelling neutron star mountains. Monthly Notices of the Royal Astronomical Society, Volume 500(4). 5570–5582, https://doi.org/10.1093/mnras/staa3635
Illustration by Dana Dumea
[…] post Bug-sized mountains that might power ripples in space-time first appeared on United Academics […]