For nearly a century, theoretical physicists have struggled to reconcile Einstein’s general relativity with quantum field theory, the framework describing subatomic particles and their interactions. Such a dream unification theory of contemporary physics is called quantum gravity.
In principle, anything that has mass or energy feels and produces gravity. There is no reason to assume that that would be any different at the atomic and subatomic levels. Searching for general relativity effects at the smallest distances possible—below the size of an atom— is fundamental to formulating consistent quantum gravity models.
Since gravity is an attractive force, the first attempt would be to propose an experiment measuring the gravitational attraction between two atoms and some materials or the Earth. The problem is that electromagnetism is the dominant force at the atomic level and the strong and electro-weak forces rule the nucleus. These forces overshadow gravity entirely, hindering observations concerning how fundamental particles experience gravity.
Many clever quantum gravity tests have been proposed, but none of them have succeeded yet.
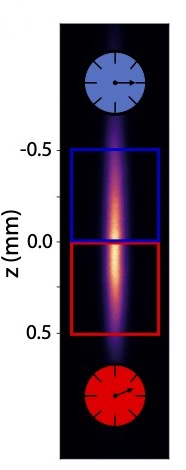
In an article published last week in Nature, Tobias Bothwell and collaborators reported having measured the dilation of time—a general relativity prediction— due to gravity in atomic clocks at an unprecedented small scale and with remarkable precision.
Time dilation means that clocks tick at different rates depending on how intense gravity is in their environment. The stronger the gravity, the slower the clock ticks. Time will go slower for a clock close to the Earth and faster for one further from the Earth.
This effect has been successfully tested over large distances. For instance, in 1971, Hafele and Keating took high precision clocks on a commercial flight, observing differences between the airplane-based clock and ground-based clock that were consistent with special and general relativity.
One prominent example that is currently in use is GPS. Because satellites are further away from the Earth than we are, their clocks tick faster than our clocks. For this reason, for GPS to be precise, computers actually need to correct time in order to compensate for this effect. The extreme case of time dilation would be a clock inside a black hole. According to general relativity, clocks close enough to a black hole would stop entirely!
Since the effect at the atomic level could be extremely small, high precision measurements are required. Here is where atomic clocks come in handy. Atomic clocks don’t need to show gravitational attraction; they just need to tell time!
How do atoms do that? Light travels in space as electromagnetic waves. The frequency of light represents the number of waves that pass through a point in one second. Each element in the periodic table (always) reacts to particular frequencies, going from a state of energy to an excited state of energy. Atomic clocks “tick” according to the microwave light frequency they need to jump to an excited state, with remarkable precision. The expected error of an atomic clock is roughly one second in every hundred million years!
However, mastering the experimental technique to use this property of atoms to measure time dilation at small scales has been extremely challenging.
Previous research led by C. W. Chou successfully measured time dilation close to the Earth using aluminum atomic clocks separated vertically by 33 cm.
In this novel research, Bothwell and his team trapped about 100,000 strontium atoms—used them as atomic clocks— in a vacuum chamber, ultra cooling them to less than one degree Celsius above the absolute zero (100 nano kelvin). They used lasers to organize the atomic clocks in a column of layers separated by one millimeter (about the thickness of a credit card). They obtained a clear gradient of tick rates, corroborating Einstein’s prediction in nature at millimeter distances.
We still don’t know the nature of time. Still, though, scientists manage to elegantly use time to test gravity with the high potential for telling us more about quantum gravity.
References
Bothwell, T., Kennedy, C. J., Aeppli, A., Kedar, D., Robinson, J. M., Oelker, E., Staron, A., & Ye, J. (2022). Resolving the gravitational redshift across a millimeter-scale atomic sample. Nature, 602(7897), 420–424. https://doi.org/10.1038/s41586-021-04349-7
Featured image: JILA researchers measured time dilation, or how an atomic clock’s ticking rate varied by elevation, within this tiny cloud of strontium atoms. Credit: JACOBSON/NIST.